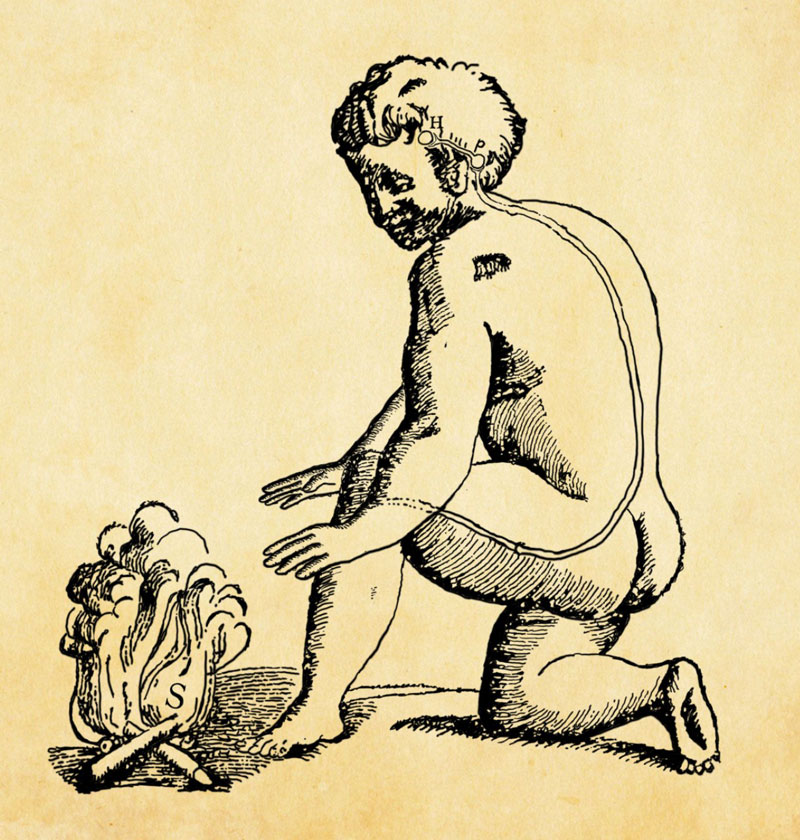
Homeostasis is a defining characteristic of life and is essential for survival and health. As a result, many of our behaviors are motivated by the need to maintain homeostasis, as seen by drinking when thirsty and stopping eating when we feel full. The Kim lab seeks to understand how sensory information regulates behaviors that maintain homeostasis, with a particular focus on brain-body communication, and to use this knowledge to develop novel therapeutic strategies for obesity and stress-related disorders.
Click here to see all publications from the Kim lab: PubMed / Google Scholar
Neural circuit mechanisms underlying homeostasis-maintaining behaviors
Currently, the majority of the lab’s research focuses on the neural mechanisms underlying ingestive and thermoregulatory behaviors, two related innate behaviors that work together to maintain energy, fluid, and thermal homeostasis. Since these well-conserved behaviors are regulated by sensory stimuli that can be precisely and quantitatively controlled in experimental settings, it is relatively facile to identify and characterize sensory afferent pathways and integration circuits in mice. Using an arsenal of circuit-interrogation techniques such as optogenetics, pharmacogenetics, two-photon Ca2+ imaging, fiber photometry, tracing and molecular tools, and surgical approaches, we have identified two key entry points for the investigation of ingestive and thermoregulatory behaviors in the recent years. Using these as entry points, we are now concentrating on defining the additional circuits components for ingestive and thermoregulatory behaviors and understanding the precise encoding of information by these neurons at the single-cell level. Our long-term research goal is to define complete circuits for ingestive and thermoregulatory behaviors from sensory origin to motor output, and to understand their interactions and context-dependent modulation, particularly under stress and disease conditions.
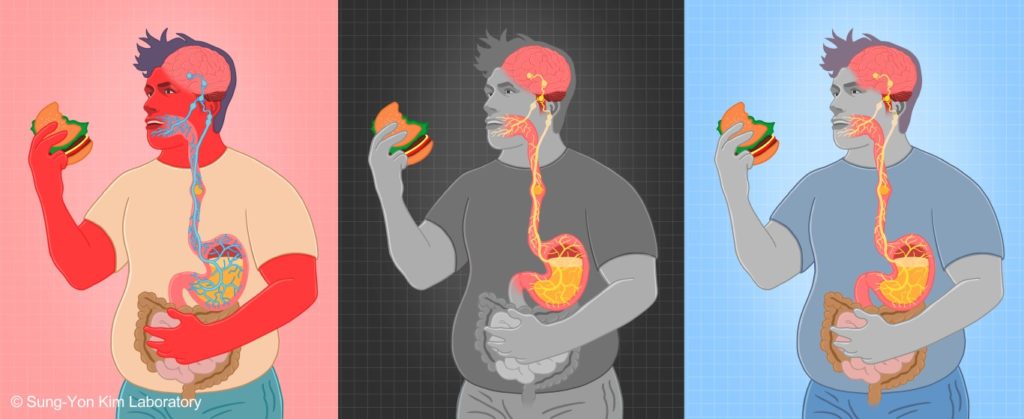
Mechanosensory feedback control of ingestion
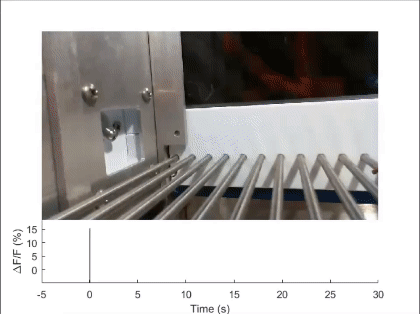
First, mechanosensory feedback from the digestive tract to the brain is critical for limiting excessive food and water intake, but the underlying gut-brain communication pathways and mechanisms remain poorly understood. We found that Pdyn+ neurons in the parabrachial nucleus monitor consumption using mechanosensory signals from the upper digestive tract (Nature 2020). Upon receiving mechanosensory signals, these neurons generate aversive and sustained appetite-suppressing signals that function as negative feedback on ingestion to prevent harmful overconsumption. We continue to investigate the origin of mechanosensory feedback signals, their gut-brain signaling pathway, and how the negative feedback signals are integrated with the need state to determine the final behavioral output — whether to stop eating or not (related reviews: Nat Rev Neurosci 2022, EMM 2022). We are also expanding our investigation into the neural mechanisms of appetite suppression induced by various external and internal stressors (related paper: EN 2022).
- Kim et al. A neural circuit mechanism for mechanosensory feedback control of ingestion. Nature 2020
_*featured as Highlight, Nature Reviews Gastroenterology & Hepatology 2020
_*selected as one of the Top 100 National Research and Development Projects in 2021
_(K-Lab News, media coverage and Sung-Yon’s talk videos) - Kim et al. Neural signalling of gut mechanosensation in ingestive and digestive processes. Nature Reviews Neuroscience 2022
_(K-Lab News) - Ahn et al. Brain circuits for homeostatic and non-homeostatic appetites. Exp Mol Med. 2022
- An et al. Lateral septum somatostatin neurons are activated by diverse stressors. Exp Neurobiol. 2022
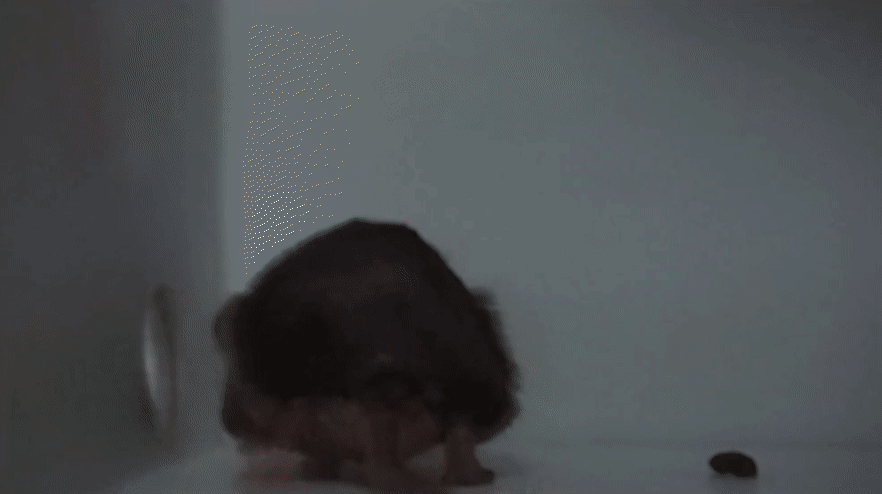
Behavioral thermoregulation
Next, thermoregulatory behavior is a basic motivated behavior for body temperature homeostasis. Despite its fundamental importance, the underlying mechanism remains strikingly unclear. We found that Vgat+ neurons in the lateral hypothalamus (LH) are generally required for diverse thermoregulatory behaviors (Neuron 2022). LH Vgat+ neurons appear to encode motivation to avoid aversive thermal stimulus, and this encoding requires thermosensory inputs from the parabrachial nucleus. This study reported the first identification of a forebrain neural substrate necessary for behavioral thermoregulation. We are now dissecting the functional heterogeneity within LH Vgat+ neurons using simultaneous multiphoton imaging and stimulation of these neurons. We are also seeking to define the thermosensory input pathways from the periphery, and using these inputs to understand how thermosensory signals are integrated in the brain to give rise to the encoding of thermal ‘reward’ and guide appropriate thermoregulatory behavior. Finally, we are examining if and how the encoding of thermosensory signals and thermoregulatory behavior are altered under disease states, such as inflammation-induced fever.
- Jung et al. A forebrain neural substrate for behavioral thermoregulation. Neuron 2022
_*featured as Previews, Neuron 2022.
_(K-Lab News, media coverage and Sung-Yon’s talk video)
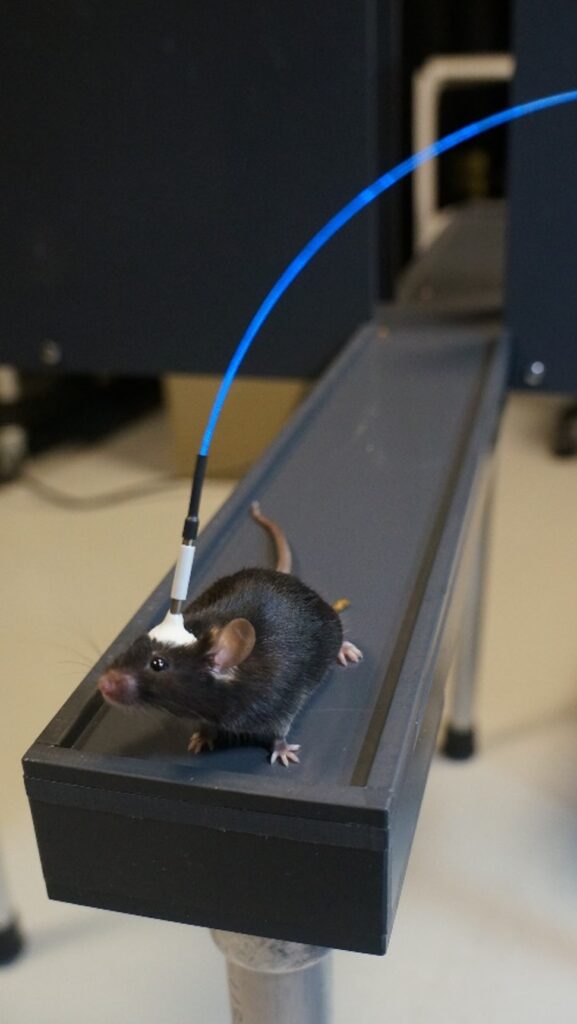
Optogenetic dissection of amygdala and extended amygdala circuits in the anxious state
Sung-Yon’s early publications identified the specific neurons and projections in the amygdala and extended amygdala that control the specific features of the anxious state, as well as the overall anxiety level. By combining optogenetics with behavioral and physiological assays, he determined the causal functions of each circuit element in anxiety-related behaviors and physiological symptoms. He also characterized their encoding of anxiogenic stimuli, synaptic connectivity, and network properties using in vivo and ex vivo electrophysiology and single-cell calcium imaging. These studies revealed that basolateral amygdala projections to both the central amygdala and the bed nucleus of the stria terminalis (BNST) reduces the overall anxiety level, that the BNST consists of at least two subnuclei (oval and anterodorsal) that oppositely regulate the level of anxiety, and that diverging pathways from the anteriodorsal BNST implement independent features of anxiolysis: reduced risk-avoidance, reduced respiratory rate, and increased positive valence (mediated by projections to the lateral hypothalamus, parabrachial nucleus, and the ventral tegmental area, respectively) (Nature 2011, Nature 2013). This body of work, which constituted Sung-Yon’s PhD thesis at Stanford, was internationally recognized by the Donald B. Lindsley Prize from the Society for Neuroscience in 2014.
- Tye*, Prakash*, Kim* et al. Amygdala circuitry mediating reversible and bidirectional control of anxiety. Nature 2011
- Kim et al. Diverging neural pathways assemble a behavioural state from separable features in anxiety. Nature 2013
_*featured as News and Views, Nature 2013
During this time, he also contributed to other studies aimed at a circuit-level understanding of anxiety and depression (Nature 2012, Nature 2013, Nature 2015) and co-authored a review of studies employing optogenetic tools to examine emotional valence and motivated behaviors (Brain Res 2013). The Kim lab continues to investigate extended amygdala circuits, with a current emphasis on how sensory information regulates innate behaviors (a related review: Mol Cells 2021).
- Warden et al. A prefrontal cortex-brainstem neuronal projection that controls response to behavioural challenge. Nature 2012
- Tye et al. Dopamine neurons modulate neural encoding and expression of depression-related behaviour. Nature 2013
- Adhikari et al. Basomedial amygdala mediates top-down control of anxiety and fear. Nature 2015
- Nieh et al. Optogenetic dissection of neural circuits underlying emotional valence and motivated behaviors. Brain Res. 2013
- Kim and Kim. Functional dissection of glutamatergic and GABAergic neurons in the bed nucleus of the stria terminalis. Mol Cells. 2021
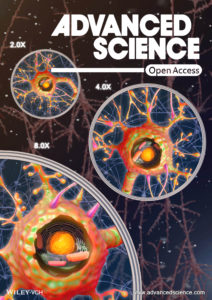
Technique development
In addition to neural circuits for the anxious state and homeostasis-maintaining behaviors, Sung-Yon and his colleagues played a prominent role in the development and application of the recently emerging ‘3D histology’ techniques. This was driven by his understanding that obtaining high-resolution structural and molecular information while preserving a global perspective is essential for comprehending the function of a system, particularly one as complex as the brain. During my PhD studies, Sung-Yon collaborated with Dr. Kwanghun Chung and contributed to the development of CLARITY, which is now a widely used tissue clearing technique (Nature 2013, Trends Cogn Sci 2013), and as a postdoctoral fellow in the Chung lab at MIT, Sung-Yon continued to develop methods for labeling large-scale tissues and sought to find a way to minimize protein loss. There, he invented a novel chemical transport concept and a technique termed stochastic electrotransport, which uses rotating electric field to rapidly disperse molecules of interest throughout large-scale tissues without distorting the sample (PNAS 2015). He also contributed to the development of a new glutaraldehyde-based fixation technique using, termed SWITCH, for preserving protein content while clearing tissue samples (Cell 2015).
- Chung et al. Structural and molecular interrogation of intact biological systems. Nature 2013
- Kim et al. Light microscopy mapping of connections in the intact brain. Trends Cogn Sci. 2013
- Kim et al. Stochastic electrotransport selectively enhances the transport of highly electromobile molecules. Proc Natl Acad Sci U S A. 2015
- Murray et al. Simple, Scalable Proteomic Imaging for High-Dimensional Profiling of Intact Systems. Cell 2015
In the Kim lab, we continue to develop and apply these 3D histology techniques. Together with a biomaterial chemist in the same department, we developed the ZOOM technique, which enables scalable and isotropic expansion of biological samples with easily tunable zoom factors (up to 8x) for super-resolution imaging with conventional light microscope (Adv Sci 2019). We demonstrated its application to mouse brain tissues as well as human postmortem tissues, bacteria, and exoskeletal C. elegans. We also devised a tissue expansion method in which the expansion ratio changes reversibly with temperature (ACS Appl Mater Interfaces 2021). Furthermore, collaborating with nanoscientists and mechanical engineers at Seoul National University, we developed 3D histology-based platforms for quantitatively analyzing nanoparticle distribution and evaluating anti-angiogenic effect in tumor vascular microenvironments (Bioconj Chem 2020, ACS Nano 2021, Acta Biomater 2022), as well as imaging pathological samples using novel citrate-based fluorophore (Sci Adv 2022). We have also wrote a review and a book chapter (Mol Cells 2016, Seo et al. 2019) aimed at disseminating the 3D histology methods to the broad community of biomedical science and enabling widespread application of our tools, and helped others in applying our techniques to the peripheral nervous system (JCI 2020) and brain organoids (Nat Comm 2021).
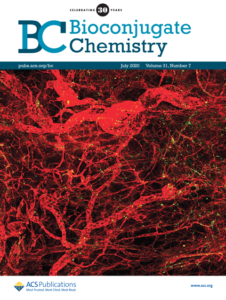
- Park et al. Scalable and isotropic expansion of tissues with simply tunable expansion ratio. Adv Sci. 2019
_*featured as the Front Cover - Kang et al. Reversible expansion microscopy using thermally adjustable expansion factor using thermoresponsive biospecimen-hydrogel hybrids. ACS Appl. Mater. Interfaces. 2021
- Koo et al. Large-scale 3D optical mapping and quantitative analysis of nanoparticle distribution in tumor vascular microenvironment. Bioconjugate Chem. 2020
_*featured as the Front Cover - Lee S, et al. 3D microfluidic platform and tumor vascular mapping for evaluating anti-angiogenic RNAi-based nanomedicine. ACS Nano. 2021
- Ahn et al. 3D microengineered vascularized tumor spheroids for drug delivery and efficacy testing. Acta Biomater. 2022
- Pac et al. Three-dimensional imaging and analysis of pathological tissue samples with de novo generation of citrate-based fluorophores. Sci Adv. 2022
- Seo et al. Clearing and Labeling Techniques for Large-Scale Biological Tissues. Mol Cells. 2016
- Seo et al. Chemical Processing of Brain Tissues for Large-Volume, High-Resolution Optical Imaging. In: Kao, Keiser, Gogoi. (eds) Advanced Optical Methods for Brain Imaging. Springer (Book chapter). 2019
- Lu et al. Tentonin 3/TMEM150C senses blood pressure changes in the aortic arch. J Clin Invest. 2020
- Cho et al. Microfluidic device with brain extracellular matrix promotes structural and functional maturation of human brain organoids. Nat Comm. 2021